As humans continue to further explore the fields of science, they deepen their understanding of convoluted subjects through the use of advanced technologies. One of the notable technologies today is the CRISPR-Cas9 system, a highly precise DNA editing tool that allows for genome manipulation in humans, animals, and plants. CRISPR (Clustered Regularly Interspaced Short Palindromic Repeats) works with a CRISPR-associated (Cas) nuclease, which was originally derived from a genome editing system used as immune defense by bacteria. To put it simply, if infected by a virus, the bacteria captures a small piece of the invading viruses’ DNA and inserts their own DNA to create a CRISPR segment (Tavakoli et al., 2021). With this segment, the bacteria can produce RNA segments that will attach to parts of the viruses’ DNA if it attacks again. The bacteria use the Cas9 enzyme to cut the viruses’ DNA apart and incapacitate it.
Scientists adapted the system so it could be inputted into the human genome and edit DNA. Researchers created small segments of RNA that have a “guide” sequence which binds to a target sequence in the DNA and attaches to the Cas9 enzyme (Tavakoli et al., 2021). The guide RNA (gRNA) identifies the intended DNA sequence and the Cas9 enzyme cuts the specific location, similar to how the process occurs in bacteria. The cell’s own DNA machinery is then used to add or delete pieces of the genetic material, known as gene knock-in and gene knockout, respectively. However, it can also alter the DNA by replacing segments with more customized sequences. CRISPR activation (CRISPRa) is used to up-regulate the expression of a gene while CRISPR interference (CRISPRi) down-regulates the expression of a cell (CRISPR Guide).
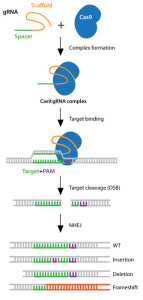
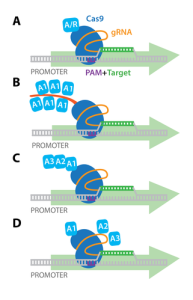
Note. Adapted from CRISPR Guide, Addgene, 2022.
There are many potential applications in cancer immunotherapy, treatment of genetic diseases, improving plant genetic variation, and more. For example, there have been advances towards mitigating the effects of blood disorders such as sickle cell disease (SCD). In those with SCD, the misshapen red blood cells can block blood vessels, thus slowing or stopping blood flow. Effects of this fatal disease are anemia, chronic pain, strokes, and organ damage. In this instance, CRISPR technology is not used to directly rectify the disease-inducing gene variant. Instead of restoring adult hemoglobin, it works to increase the levels of fetal hemoglobin, an oxygen carrier protein that only fetuses make in the womb (Henderson, 2022). Since it’s not affected by the sickle cell mutation, it can be a substitute for the defective adult hemoglobin in red blood cells (Henderson, 2022).
Note. Adapted from CRISPR Clinical Trials: A 2022 Update, Henderson, 2022, Copyright 2022 by Innovative Genomics Institute.
As seen in the diagram above, SCD symptoms begin during infancy when levels of fetal hemoglobin decrease. The blood stem cells are harvested from the blood and then the genomes are edited to activate the fetal hemoglobin gene. Next, chemotherapy eliminates the disease-inducing blood stem cells from the person’s body, and the genome-edited stem cells are put back into the bloodstream through an IV, creating a new blood stem cell population that produces fetal hemoglobin (Henderson, 2022). This technique is ex vivo genome editing, where the editing occurs outside the body to prevent the risk of lingering and unwanted CRISPR components in the body. Those treated for SCD in CRISPR clinical trials show normal to near-normal hemoglobin levels with at least 30% as fetal hemoglobin (Henderson, 2022).
It raises the following questions: is it possible to select certain features in humans that are seen as favorable or desirable? Is it possible to have almost complete manipulation of physical features or to enhance the body’s biological processes to unlock superhuman abilities only seen in movies? These human experimentations would be best measured if implemented during the early stages of life so as to let the body integrate the changes and develop properly. Perhaps we could alter ourselves to increase muscle mass, heighten athletic ability, or augment intelligence. In regard to altering the human genome, there are certain limitations as to what can and can’t be done without violating certain ethical boundaries.
This can be seen in the case of He Jianku, a Chinese scientist who used CRISPR to edit two embryos, both of which are now living babies. Though there was little information that only came from He himself and the Chinese government, it has been said that sometime in late 2017, He injected the two embryos with a CRISPR construct that would delete a 32-base-pair in the CCR5 gene on chromosome 3, leading to a non-functional CCR5 protein (Greely, 2019). He sought to achieve humans who could not contract AIDS because they don’t have this functioning protein (Greely, 2019). Though this theory was later proved wrong and the CRISPR construct did not fulfill his intentions, he managed to induce never before seen changes that led to the production of non-functional proteins. His actions prompted the Chinese court to convict him on the basis of deliberately violating medical regulations (Greely, 2019). Could it be argued that pushing the boundaries are necessary for scientific growth and greater human evolution? How far is too far? As CRISPR and other gene editing tools continue to develop, we can keep imagining the unnerving yet exciting possibilities that are awaiting.
Literature Cited
CRISPR Guide. (n.d.). Addgene.
Greely, H. T. (2019). CRISPR’d babies: human germline genome editing in the ‘He Jiankui affair’. Journal of Law and Biosciences, 6(1), 111-183.
Henderson, H. (2022). CRISPR Clinical Trials: A 2022 Update. Innovative Genomics Institute.
Tavakoli, K., Pour-Aboughadareh, A., Kianersi, F., Poczai, P., Etminan, A., & Shooshtari, L. (2021). Applications of CRISPR-Cas9 as an Advanced Genome Editing System in Life Sciences. BioTech, 10(3), 14.
What are genome editing and CRISPR-Cas9? (2022). MedlinePlus.